
This is due to directing effects of substituents in conjugation with the benzene ring. Some electron donating groups have an extra resonance form in which there is a double bond between the atom and the carbon on the benzene. The reason for this can be explained by the different carbocation resonance structures of the ortho, meta and para positions. Examples of electron donating groups: -CH 3, -OCH 3, -OH, -NH 2Įlectron donating groups cause the second subtituent to add on to the para or ortho position on the benzene ring. Electron donating groups are donating by induction (Activating and Deactivating Benzene Rings) and resonance.
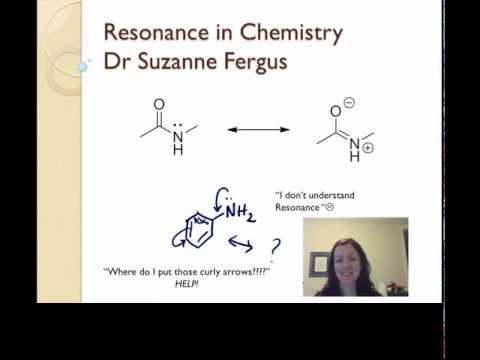
Electron donating groups are alkyl groups, phenyl groups or substituents that have a lone pair of electrons on the atom directly bonded to the ring. The first scenario for adding an electrophile to a monosubstituted benzene ring is when the substituent is an electron donating group. The three general positions of a disubstituted benzene ring are ortho, meta and para.įi gure 1: The Effect of an Electron Donating Groups on a Benzene Ring structures that have the same energy as each other.įinally, see how structure II leaves carbon without an octet? You may have learned that atoms prefer to satisfy the octet rule.īecause of a lack of that here, structure II is the most minor resonance contributor (least stable) of these four.\) Those would be known as degenerate structures, i.e. That automatically means III and IV are the minor resonance contributors (second-most stable).Īlso, notice how structures III and IV are actually identical just reflect them along a vertical axis and they are the same. That means I is the major resonance contributor. We start at I and continuously move clockwise.įirst, see how structures III and IV do not have minimized formal charges? Although there is net charge cancellation, nitrogen, being less electronegative than oxygen, tends less to pull electrons towards it therefore, the major (most stable) resonance structure gives the most electron density to oxygen. The curved arrows indicate the movement of electrons that lead to a new resonance contributor. Let's suppose that we examine the resonance structures of urea, #"H"_2"NCONH"_2#. The most stable structural "snapshots" of a molecule's electron distribution is its major resonance structure. So overall, that's why resonance structures that represent the most stable state of a molecule are the ones that occur most often. To achieve the smallest amount of water in multiple glasses, you should get the same amount in all glasses, not pour it all into one glass. You can also analogize electron delocalization with glasses of water.
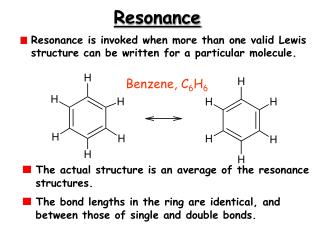
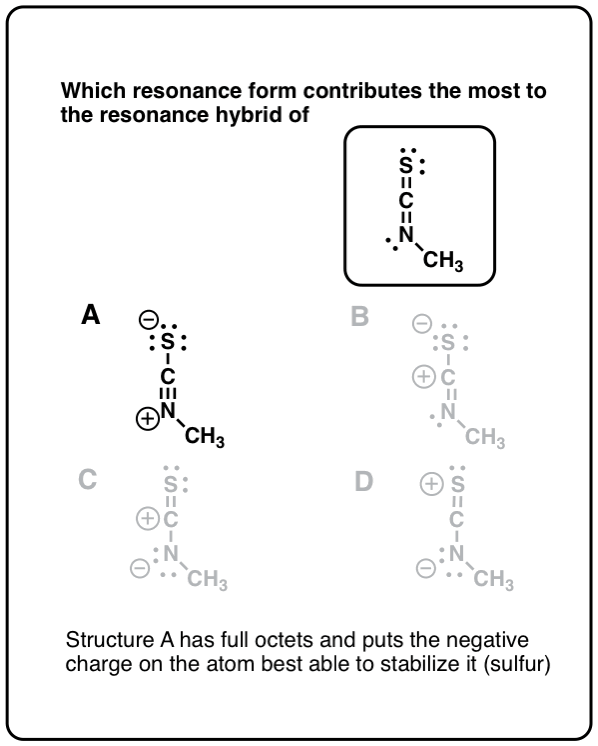
The more room (orbitals) the electrons have available to move, the more distributed their kinetic energy can be, and in some sense, the less energy "buildup" there would be in select orbitals. Similarly, molecules don't want to be overly excited/hyper, and instead want to achieve the minimum energy, or ground-state energy.ĭelocalizing the electrons in a system with many #pi# electrons helps make that happen in molecules that we draw as resonance structures. So, you drink the minimum amount of coffee so you can just stay awake. If you drink too much coffee in the morning, you might get too hyper over the course of the day, and I don't think anyone really wants to be overly hyper. Minimum energy is analogous to not drinking too much coffee in the morning.
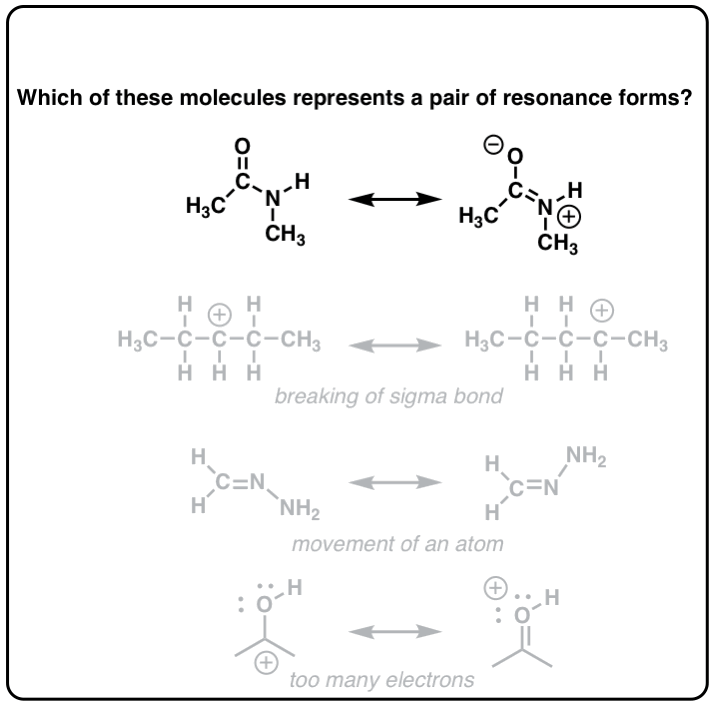
Molecules always strive for achieving the minimum energy, whether through electronic relaxations, electron delocalization, or other processes. It's not that certain resonance structures are stable because they occur most often, but that the resonance structures that represent the most stable state of a molecule occur most often. We need to be careful of the cause/effect of this.
